Shigella and Shigellosis (page 1)
This chapter has 4 pages
Shigella is a genus of gamma proteobacteria in the family Enterobacteriaceae.Shigellae are Gram-negative, nonmotile, non-spore forming, rod-shaped bacteria, very closely related to Escherichia coli.
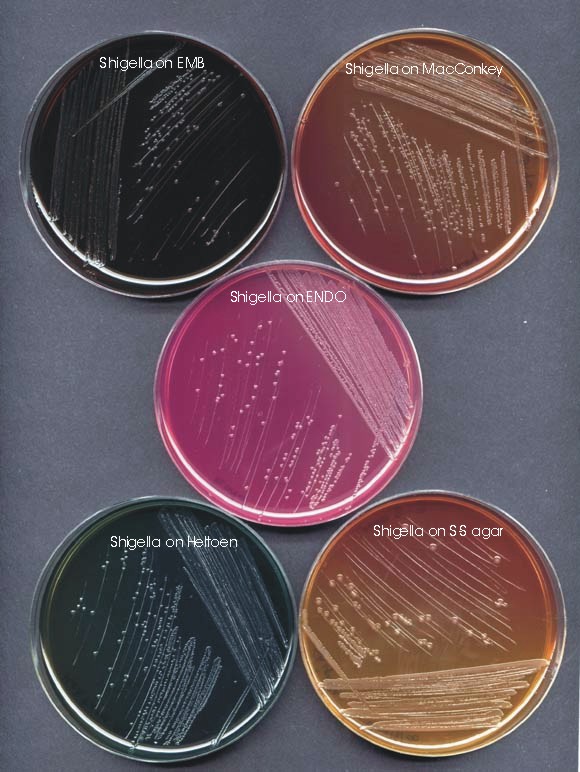
Figure 1. Several media have been designed to selectively grow enteric bacteria and allow differentiation of Salmonella and Shigella from E. coli. The primary plating media shown here are eosin methylene blue (EMB) agar, MacConkey agar, ENDO agar, Hektoen enteric (HE) agar and Salmonella-Shigella (SS) agar.
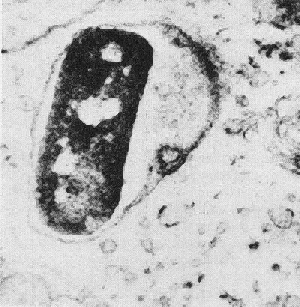
Figure 2. Electron Micrograph of Shigella in a membrane-enclosed endosome of an epithelial cell

END OF CHAPTER
This chapter has 4 pages
Shigella is a genus of gamma proteobacteria in the family Enterobacteriaceae.Shigellae are Gram-negative, nonmotile, non-spore forming, rod-shaped bacteria, very closely related to Escherichia coli.
Shigellosis
Shigellosis is an infectious disease caused by various species of Shigella. People infected with Shigella develop diarrhea, fever and stomach cramps starting a day or two after they are exposed to the bacterium. The diarrhea is often bloody. Shigellosis usually resolves in 5 to 7 days, but in some persons, especially young children and the elderly, the diarrhea can be so severe that the patient needs to be hospitalized. A severe infection with high fever may also be associated with seizures in children less than 2 years old. Some persons who are infected may have no symptoms at all, but may still transmit the bacteria to others.
Shigellosis is an infectious disease caused by various species of Shigella. People infected with Shigella develop diarrhea, fever and stomach cramps starting a day or two after they are exposed to the bacterium. The diarrhea is often bloody. Shigellosis usually resolves in 5 to 7 days, but in some persons, especially young children and the elderly, the diarrhea can be so severe that the patient needs to be hospitalized. A severe infection with high fever may also be associated with seizures in children less than 2 years old. Some persons who are infected may have no symptoms at all, but may still transmit the bacteria to others.
Shigella were discovered over 100 years ago by the Japanese microbiologist, Shiga, for whom the genus is named. There are four species of Shigella: S. boydii, S. dysenteriae, S. flexneri, and S. sonnei. Shigella sonnei, also known as Group D Shigella, accounts for over two-thirds of the shigellosis in the United States. Shigella flexneri, or Group B Shigella, accounts for almost all of the rest. Other types of Shigella are rare in this country, although they are important causes of disease in the developing world. One type, Shigella dysenteriae type 1, causes deadly epidemics in many developing regions and nations.
Diagnosis
Determining that Shigella is the cause of the illness depends on laboratory tests that identify the bacteria in the stool of an infected person. Some of the tests may not be performed routinely, so the bacteriology laboratory should be instructed to look for the organism. The laboratory can also do tests to determine which type of Shigella is involved, and which antibiotics, if any, would be best for treatment.
Determining that Shigella is the cause of the illness depends on laboratory tests that identify the bacteria in the stool of an infected person. Some of the tests may not be performed routinely, so the bacteriology laboratory should be instructed to look for the organism. The laboratory can also do tests to determine which type of Shigella is involved, and which antibiotics, if any, would be best for treatment.
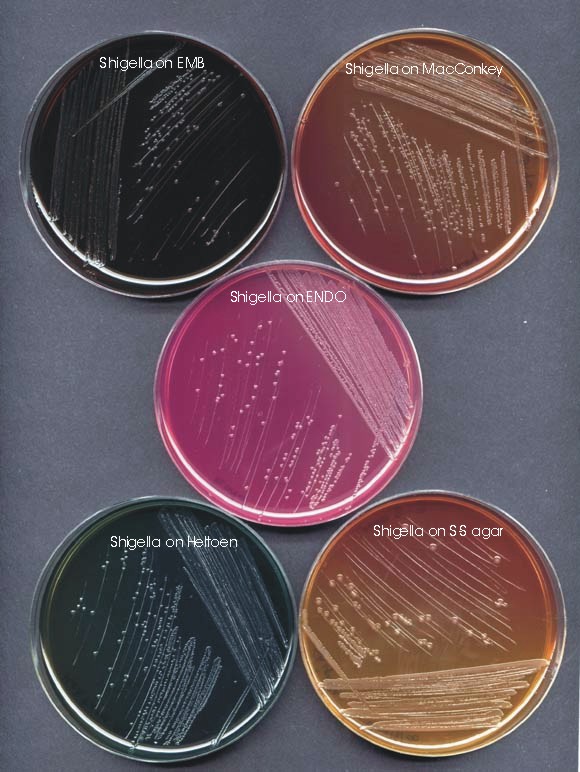
Figure 1. Several media have been designed to selectively grow enteric bacteria and allow differentiation of Salmonella and Shigella from E. coli. The primary plating media shown here are eosin methylene blue (EMB) agar, MacConkey agar, ENDO agar, Hektoen enteric (HE) agar and Salmonella-Shigella (SS) agar.
Treatment
Shigellosis can usually be treated with antibiotics. The antibiotics commonly used are ampicillin, trimethoprim/sulfamethoxazole (also known as Bactrim or Septra), nalidixic acid and the fluoroquinolone, ciprofloxacin. Appropriate treatment kills the bacteria present in the gastrointestinal tract and shortens the course of the illness.
Shigellosis can usually be treated with antibiotics. The antibiotics commonly used are ampicillin, trimethoprim/sulfamethoxazole (also known as Bactrim or Septra), nalidixic acid and the fluoroquinolone, ciprofloxacin. Appropriate treatment kills the bacteria present in the gastrointestinal tract and shortens the course of the illness.
Some Shigella have become resistant to antibiotics and inappropriate use of antibiotics to treat shigellosis can make the organisms more resistant in the future. Persons with mild infections will usually recover quickly without antibiotic treatment. Therefore, when many persons in a community are affected by shigellosis, antibiotics are sometimes used selectively to treat only the more severe cases. Antidiarrheal agents such as loperamide (Imodium) or diphenoxylate with atropine (Lomotil) are likely to make the illness worse and should be avoided.
Reiter's syndrome
Persons with diarrhea usually recover completely, although it may be several months before their bowel habits are entirely normal. About 3% of persons who are infected with Shigella flexneri may subsequently develop pains in their joints, irritation of the eyes, and painful urination. This condition is calledReiter's syndrome. It can last for months or years, and can lead to chronic arthritis which is difficult to treat. Reiter's syndrome is a late complication of S. flexneri infection, especially in persons with a certain genetic predisposition, namely HLA-B27. [Human Leukocyte Antigen B27 (HLA-B27) is a class I surface antigen in the major histocompatibility complex (MHC) on chromosome 6 and presents microbial antigens to T-cells. HLA-B27 has been strongly associated with a certain set of autoimmune diseases referred to as the "seronegative spondyloarthropathies".]
Persons with diarrhea usually recover completely, although it may be several months before their bowel habits are entirely normal. About 3% of persons who are infected with Shigella flexneri may subsequently develop pains in their joints, irritation of the eyes, and painful urination. This condition is calledReiter's syndrome. It can last for months or years, and can lead to chronic arthritis which is difficult to treat. Reiter's syndrome is a late complication of S. flexneri infection, especially in persons with a certain genetic predisposition, namely HLA-B27. [Human Leukocyte Antigen B27 (HLA-B27) is a class I surface antigen in the major histocompatibility complex (MHC) on chromosome 6 and presents microbial antigens to T-cells. HLA-B27 has been strongly associated with a certain set of autoimmune diseases referred to as the "seronegative spondyloarthropathies".]
Hemolytic Uremic Syndrome (HUS)
Hemolytic uremic syndrome (HUS) can occur after S. dysenteriae type 1 infection. Convulsions may occur in children; the mechanism may be related to a rapid rate of temperature elevation or metabolic alterations, and is associated with the production of the Shiga toxin, which is discussed below.
Hemolytic uremic syndrome (HUS) can occur after S. dysenteriae type 1 infection. Convulsions may occur in children; the mechanism may be related to a rapid rate of temperature elevation or metabolic alterations, and is associated with the production of the Shiga toxin, which is discussed below.
Transmission
Shigella is transmitted from an infected person to another usually by a fecal-oral route. Shigella are present in the diarrheal stools of infected persons while they are ill and for a week or two afterwards. Most Shigella infections are the result of the bacterium passing from stools or soiled fingers of one person to the mouth of another person. This happens when basic hygiene and handwashing habits are inadequate. It is particularly likely to occur among toddlers who are not fully toilet-trained. Family members and playmates of such children are at high risk of becoming infected. The spread of Shigella from an infected person to other persons can be stopped by frequent and careful handwashing with soap, practiced by all age groups.
Part of the reason for the efficiency of transmission is because a very small inoculum (10 to 200 organisms) is sufficient to cause infection. As a result, spread can occur easily by the fecal-oral route and readily occurs in settings where hygiene is poor.
Epidemics may be foodborne or waterborne. Shigella infections may be acquired from eating food that has become contaminated by infected food handlers. Vegetables can become contaminated if they are harvested from a field with contaminated sewage or wherein infected field workers defecate. Shigella can also be transmitted by flies. Flies can breed in infected feces and then contaminate food. Shigella infections can be acquired by drinking or swimming in contaminated water. Water may become contaminated if sewage runs into it, or even if someone with shigellosis swims or bathes or, worse, defecates, in it.
Epidemics may be foodborne or waterborne. Shigella infections may be acquired from eating food that has become contaminated by infected food handlers. Vegetables can become contaminated if they are harvested from a field with contaminated sewage or wherein infected field workers defecate. Shigella can also be transmitted by flies. Flies can breed in infected feces and then contaminate food. Shigella infections can be acquired by drinking or swimming in contaminated water. Water may become contaminated if sewage runs into it, or even if someone with shigellosis swims or bathes or, worse, defecates, in it.
Immunity and Vaccines
Once someone has had shigellosis, they are not likely to get infected with that specific type again for at least several years. However, they can still get infected with other types of Shigella. Presumably, this immunity is due to secretory IgA. Circulating antibodies can also be detected in immune individuals. Although CMI may not be ruled out, the cellular immune response is ineffective againstShigella in animal models, and Shigella-specific cytotoxic T lymphocytes have not been isolated from convalescent individuals.
In addition, factors that permit the bacterium to optimize its lifestyle in the human colon may also have been acquired by means of horizontal gene transmission from other enteric bacteria in the colon after acquisition of the prototypic virulence plasmid. An example of this is the acquisition by horizontal transfer of O-antigen genes, such as those present on the virulence plasmid ofS. sonnei, and subsequent inactivation of native O-antigen genes (30). Serotypic diversity due to the variations in O antigen is seen among Shigella strains. Such diversity likely facilitates evasion of the host humoral immune response.
Studies are underway around the world to develop a vaccine to prevent shigellosis. Since the virulence of Shigella is well-understood, and considering the present art of vaccine development, it seems that vaccination should be feasible. The need of the vaccine is based on the burden of disease globally: there are 160 million cases of shigellosis in the world each year, resulting in about 1.5 million deaths. Three approaches to shigella vaccine development that are under active investigation are: 1) parenteral O-specific polysaccharide conjugate vaccines; 2) nasal proteosomes delivering Shigella LPS; and 3) live, attenuated invasive shigella deletion mutants that are administered orally.
Several live attenuated Shigella vaccines of different serotypes have been shown to be safe, immunogenic, and in one case, effective against challenge with virulent strains. The ability to invade epithelial cells remains critical for the success of these vaccine candidates. Live, orally administered Shigella vaccine derivatives are also being evaluated as multivalent mucosal vaccines able to deliver bacterial antigens to the gut associated lymphoid tissues (GALT).
Incidence and Risk of Infection
In the United States, there are approximately 14,000 laboratory-confirmed cases of shigellosis and an estimated 448,240 total cases (85% due to S. sonnei) that occur each year, according to CDC. Groups at increased risk of shigellosis include children in child-care centers and persons in custodial institutions, where personal hygiene is difficult to maintain.
In the developing world, S. flexneri predominates. Epidemics of S. dysenteriaetype 1 have occurred in Africa and Central America with case fatality rates of 5-15%.
Pathogenesis of Shigella flexneri
Shigella flexneri causes bacillary dysentery, the symptoms of which include abdominal pain, diarrhea, fever, vomiting and blood or mucus in the stool. The bacteria are transmitted by the fecal-oral route, and through contaminated food and water. Once ingested, the bacteria survive the gastric environment of the stomach and move on to the large intestine. There, they attach to and penetrate the epithelial cells of the intestinal mucosa. After invasion, they multiply intracellularly and spread to neighboring epithelial cells, resulting in tissue destruction and characteristic pathology of shigellosis.
Entry of Shigella flexneri into Epithelial Cells
In order for S. flexneri to enter an epithelial cell, the bacterium must first adhere to its target cell. It is then internalized by a process which is similar to the mechanism of phagocytosis. Generally, the bacterium adheres to the membrane of the cell and is internalized via an endosome, which it subsequently lyses to gain access to the cytoplasm where multiplication occurs.
In order for S. flexneri to enter an epithelial cell, the bacterium must first adhere to its target cell. It is then internalized by a process which is similar to the mechanism of phagocytosis. Generally, the bacterium adheres to the membrane of the cell and is internalized via an endosome, which it subsequently lyses to gain access to the cytoplasm where multiplication occurs.
To aid its entry into the epithelial cell, the bacterial DNA encodes a number of plasmid and chromosomal proteins. These proteins are the invasion plasmid antigens (Ipa), surface presentation antigens (Spa), membrane excretion proteins (Mxi), and virulence proteins (Vir).
When the bacterium grows at 37oC, the virulence protein VirF induces the expression of the VirB protein. The VirB protein then activates the ipa, mxi, andspa promoters leading to expression of the spa and mxi operons. This results in the synthesis and assembly of a protein complex called the Mxi-Spa translocon. When the bacterium makes contact with the epithelial cell membrane, the translocon becomes activated and secretes the pre-synthesized Ipa proteins. IpaB, IpaC and IpaA associate to form a complex which interacts with the host epithelial cell membrane to induce a cascade of cellular signals which will lead to the internalization of the bacterium via an endosome. The Ipa proteins are also required for escape from the endosome.
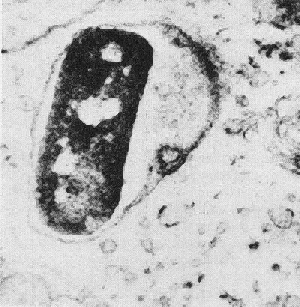
Figure 2. Electron Micrograph of Shigella in a membrane-enclosed endosome of an epithelial cell
Intracellular and Intercellular Spread
Extracellular S. flexneri cells are nonmotile, but intracellular bacteria move to occupy the entire cytoplasm of the infected cell, and they are able to spread between cells. The genes necessary for intracellular and intercellular spreading are virG (icsA) and icsB.
Extracellular S. flexneri cells are nonmotile, but intracellular bacteria move to occupy the entire cytoplasm of the infected cell, and they are able to spread between cells. The genes necessary for intracellular and intercellular spreading are virG (icsA) and icsB.
After entry into the cell, intracellular movement occurs if the bacterium expresses both an Olm ("organelle-like movement") phenotype and an alternative Ics phenotype. The expression the Olm phenotype allows the bacteria to "slide" along actin stress cables inside the host cell, while the expression of the Ics phenotype allows the bacteria to "spread" or infect adjacent cells.
Specifically, movement of S. flexneri between adjacent cells is mediated via the product of the virG (icsA) gene. The icsA gene elicits actin polymerization at the poles of the bacteria and induces the formation of protrusions. In some instances, these tightly packed actin filaments appear to form a cylinder. The bacteria in the protrusions can move through the host cell and penetrate into an adjacent cell without coming in contact with the extracellular medium where they would be rendered nonmotile.
The mxiG gene is required for Ipa protein secretion, and is also essential for entry. This gene and others in the Mxi-Spa translocon are also required for intercellular dissemination.
Pathological Effects
Following host epithelial cell invasion and penetration of the colonic mucosa,Shigella infection is characterized by degeneration of the epithelium and inflammation of the lamina propria. This results in desquamation and ulceration of the mucosa, and subsequent leakage of blood, inflammatory elements and mucus into the intestinal lumen. Patients suffering from Shigella infection will therefore pass frequent, scanty, dysenteric stool mixed with blood and mucus, since, under these conditions, the absorption of water by the colon is inhibited. This is in opposition to the diarrheal symptoms seen in patients suffering from extensive Shigella colitis, and the pathologic basis for this is unknown. It is possible that prostaglandin interactions induced by the inflammatory response to bacterial invasion contribute to diarrhea in patients with Shigella colitis.
Following host epithelial cell invasion and penetration of the colonic mucosa,Shigella infection is characterized by degeneration of the epithelium and inflammation of the lamina propria. This results in desquamation and ulceration of the mucosa, and subsequent leakage of blood, inflammatory elements and mucus into the intestinal lumen. Patients suffering from Shigella infection will therefore pass frequent, scanty, dysenteric stool mixed with blood and mucus, since, under these conditions, the absorption of water by the colon is inhibited. This is in opposition to the diarrheal symptoms seen in patients suffering from extensive Shigella colitis, and the pathologic basis for this is unknown. It is possible that prostaglandin interactions induced by the inflammatory response to bacterial invasion contribute to diarrhea in patients with Shigella colitis.
The Large Virulence Plasmid of Shigella flexneri
All virulent strains of Shigella flexneri possess a large 220kb plasmid that mediates its virulence properties. This so-called the invasion plasmid has been shown to encode the genes for several aspects of Shigella virulence, including
- Adhesins that are involved in the adherence of bacteria onto the surface of target epithelial cells
- The production of invasion plasmid antigens (Ipa) that have a direct role in theShigella invasion process
- Transport or processing functions that ensure the correct surface expression of the Ipa proteins
- The induction of endocytic uptake of bacteria and disruption of endocytic vacuoles
- The intra- and inter-cellular spreading phenotypes
- The regulation of plasmid-encoded vir genes
The presence of this plasmid was discovered in the 1980s, after the observation that essentially the entire chromosome of S. flexneri could be transferred to E. coli without reconstituting the virulence phenotype of the donor. However, the ability to invade tissue culture cells was transferred to E. coli by the conjugal mobilization of this plasmid from S. flexneri. (see below)

Figure 3. Circular map of the large virulence plasmid of Shigella. Outer ring depicts ORFs and their orientations, color coded according to functional category: 1. identical or essentially identical to known virulence-associated proteins (red); 2, homologous to known pathogenesis-associated proteins (pink); 3. highly homologous to IS elements or transposases (blue); 4. weakly homologous to IS elements or transposases (light blue); 5. homologous to proteins involved in replication, plasmid maintenance, or other DNA metabolic functions (yellow); 6. no significant similarity to any protein or ORF in the database (brown); 7. homologous or identical to conserved hypothetical ORFs, i.e., proteins of unknown function (orange); and 8. Tn501 insertion-associated genes (green). The second ring shows complete IS elements. The third ring graphs G+C content, calculated for each ORF and plotted around the mean value for all ORFs, with each value color coded for the corresponding ORF. Scale is in base pairs. The figure was generated by Genescene (DNASTAR). Venkatesan, M.M., et al. Complete DNA Sequence and Analysis of the Large Virulence Plasmid of Shigella flexneri. Infect Immun. 2001 May; 69(5): 3271�3285. http://www.pubmedcentral.nih.gov/articlerender.fcgi?artid=98286
The invasion locus on the virulence plasmid of Shigella is a pathogenicity island-like cluster that consists of 38 ORFs of the ipa-mxi-spa operons within a stretch of 32 kb of the plasmid. Genes within this locus are critical for Shigella invasion of mammalian cells, although certain genes outside this region are required for optimal invasion of tissue culture cells.
Gene | Protein Product MW | Regulatory or effector function |
virF | 30 kDa | positive regulators of the virG and ipa-mxi-spa loci |
invA(mxiB) | 38 kDa | Necessary for invasion (orients ipa gene products in outer membrane |
mxiA | 76 kDA | Same as above |
ippI | 18 kDa | Same as above |
ipaB | 62 kDa | Necessary for invasion: mediates endocytic uptake of shigellae |
ipaC | 43 kDa | Same as above |
ipaA | 38 kDa | Same as above |
ipaD | 78 kDa | Not necessary for invasion (role unknown) |
virB | 33 kDa | positive regulator of the virG and ipa-mxi-spa loci |
virG (icsA) | 120 kDa | assembles actin tails that propel the bacteria through the cell cytoplasm and into adjacent cells |
ipaH | 60 kDa | has 5 alleles; IpaH7.8 facilitates the escape of Shigella from phagocytic vacuoles |
shET2 | 60kDa | ShET2 enterotoxin |
Evolution of the Shigella virulence plasmid
Recent genetic analyses suggest that shigellae do not constitute a distinct genus as traditionally believed but rather are within the genus of E. coli, much like the enteric pathogenic E. coli. These analyses indicate that Shigella emerged from E. coli seven or eight independent times during evolution, leading to three clusters of Shigella, each of which contains serotypes from multiple traditional species, and four or five additional forms, each of which contains one traditional serotype. The three main Shigella clusters are estimated to have evolved 35,000 to 270,000 years ago, which predates the development of agriculture and makes shigellosis one of the early infectious diseases of humans.
Recent genetic analyses suggest that shigellae do not constitute a distinct genus as traditionally believed but rather are within the genus of E. coli, much like the enteric pathogenic E. coli. These analyses indicate that Shigella emerged from E. coli seven or eight independent times during evolution, leading to three clusters of Shigella, each of which contains serotypes from multiple traditional species, and four or five additional forms, each of which contains one traditional serotype. The three main Shigella clusters are estimated to have evolved 35,000 to 270,000 years ago, which predates the development of agriculture and makes shigellosis one of the early infectious diseases of humans.
The defining event each time Shigella arose was almost certainly the acquisition of an historical precursor of the current-day virulence plasmid. The data also suggest that the loss of specific catabolic pathways (inability to utilize lactose and mucate and to decarboxylate lysine), loss of motility, and expansion of O-antigen diversity that are characteristic of Shigella strains occurred more recently than the acquisition of the plasmid.
Since the plasmid was acquired at distinct times, one would predict that differences reflecting the evolution of the plasmid could be obtained by genetic comparison of virulence plasmids of the seven different Shigella evolutionary groups. Subsequent to the acquisition of the virulence plasmid, divergence ofShigella clones from E. coli would involve clonal divergence (accumulation of mutations by base substitution), horizontal transfer of genetic material from other species, and loss of gene sequences that interfere with pathogenicity.
Certain horizontal gene transfer events have been key to the evolution ofShigella. A quintessential feature of Shigella is its ability to invade mammalian cells and access the cell cytoplasm, defining a niche unique among enteric Gram-negative bacteria, with the exception of enteroinvasive E. coli. Thus, the acquisition and evolution of the ipa-mxi-spa pathogenicity island, which encodes all of the genes required for cell invasion and phagolysosomal lysis, permitted a major alteration in pathogenesis. Likewise, the acquisition of virG (icsA), which mediates actin assembly on Shigella, and virF and virB, the regulators of the virGand ipa-mxi-spa loci, were key to the emergence of Shigella. Since all Shigellaserotypes contain these loci, they were probably all present on the prototypic virulence plasmid.
The Shiga Toxin
The Shiga toxin, also called the verotoxin, is produced by Shigella dysenteriaeand
enterohemorrhagic Escherichia coli (EHEC), of which the strain O157:H7 has become the best known.
enterohemorrhagic Escherichia coli (EHEC), of which the strain O157:H7 has become the best known.
The syndromes associated with shiga toxin include dysentery, hemorrhagic colitis, and hemolytic uremic syndrome. The name is dependent upon the causative organism and the symptoms, which may include severe diarrhea, abdominal pain, vomiting, and bloody urine (in the case of hemolytic uremic syndrome).
The onset of symptoms is generally within a few hours, with higher doses leading to more rapid onset. There is no antidote for the toxin. Supportive care requires maintenance of fluid and electrolyte levels, and monitoring and support of kidney function.
Immunoassays are available for rapid diagnosis of the toxin.
Inactivation of the toxin is achieved by steam treatment, oxidizing agents such as bleach, and chemical sterilizing agents such as glutaraldehyde.
The toxicity of Shiga Toxin for the mouse (LD50) is <20 micrograms/kg by intravenous or intraperitoneal administration. There is no published data on the inhalation toxicity of Shiga toxin. However, there are often indirect effects on the lungs when the toxin is taken in as a food contaminant.
Table 2. The toxin has been given several trivial names depending on the bacterium that produces it and the gene that encodes it.
|
Structure of the Toxin
The toxin has a molecular weight of 68,000 da. It is a multi-subunit protein made up one molecule of an A subunit (32,000 molecular weight) responsible for the toxic action of the protein, and five molecules of the B subunit (7,700 molecular weight) responsible for binding to a specific cell type.
The toxin has a molecular weight of 68,000 da. It is a multi-subunit protein made up one molecule of an A subunit (32,000 molecular weight) responsible for the toxic action of the protein, and five molecules of the B subunit (7,700 molecular weight) responsible for binding to a specific cell type.
Mechanism of Action
The toxin acts on the lining of the blood vessels, the vascular endothelium. The B subunits of the toxin bind to a component of the cell membrane known as Gb3 and the complex enters the cell. When the protein is inside the cell, the A subunit interacts with the ribosomes to inactivate them. The A subunit of Shiga toxin is an N-glycosidase that modifies the RNA component of the ribosome to inactivate it and so bring a halt to protein synthesis leading to the death of the cell. The vascular endothelium has to continually renew itself, so this killing of cells leads to a breakdown of the lining and to hemorrhage. The first response is commonly a bloody diarrhea. This is because Shiga toxin is usually taken in with contaminated food or water.
The toxin acts on the lining of the blood vessels, the vascular endothelium. The B subunits of the toxin bind to a component of the cell membrane known as Gb3 and the complex enters the cell. When the protein is inside the cell, the A subunit interacts with the ribosomes to inactivate them. The A subunit of Shiga toxin is an N-glycosidase that modifies the RNA component of the ribosome to inactivate it and so bring a halt to protein synthesis leading to the death of the cell. The vascular endothelium has to continually renew itself, so this killing of cells leads to a breakdown of the lining and to hemorrhage. The first response is commonly a bloody diarrhea. This is because Shiga toxin is usually taken in with contaminated food or water.
The toxin is effective against small blood vessels, such as found in the digestive tract, the kidney, and lungs, but not against large vessels such as the arteries or major veins. A specific target for the toxin appears to the vascular endothelium of the glomerulus. This is the filtering structure that is a key to the function of the kidney. Destroying these structures leads to kidney failure and the development of the often deadly and frequently debilitating hemolytic uremic syndrome. Food poisoning with Shiga toxin often also has effects on the lungs and the nervous system.
Shiga Toxin-Producing Escherichia coli (STEC)
Shiga toxin-producing Escherichia coli is a type of enterohemorrhagic E. coli(EHEC) bacteria that can cause illness ranging from mild intestinal disease to severe kidney complications. Enterohemorrhagic E. coli include the relatively important serotype E. coli O157:H7, but other non-O157 strains, such as O111 and O26, have been associated with shiga toxin production.
Shiga toxin-producing Escherichia coli is a type of enterohemorrhagic E. coli(EHEC) bacteria that can cause illness ranging from mild intestinal disease to severe kidney complications. Enterohemorrhagic E. coli include the relatively important serotype E. coli O157:H7, but other non-O157 strains, such as O111 and O26, have been associated with shiga toxin production.
The incubation period for STEC ranges from 1 to 8 days, though typically it is 3 to 5 days. Typical symptoms include severe abdominal cramping, sudden onset of watery diarrhea, frequently bloody, and sometimes vomiting and a low-grade fever. Most often the illness is mild and self-limited generally lasting 1-3 days. However, serious complications such as hemorrhagic colitis, Hemolytic Uremic Syndrome (HUS), or postdiarrheal thrombotic thrombocytopenic purpura (TTP) can occur in up to 10% of cases.
Cases and outbreaks of Shiga toxin-producing Escherichia coli have been associated with the consumption of undercooked beef (especially ground beef), raw milk, unpasteurized apple juice, contaminated water, red leaf lettuce, alfalfa sprouts, and venison jerky. The bacteria have also been isolated from poultry, pork and lamb. Person-to-person spread via fecal-oral transmission may occur in high-risk settings like day care centers and nursing homes.
Although anyone can get infected, the highest infection rates are in children under age 5. Elderly patients also account for a large number of cases. Outbreaks have occurred in child-care facilities and nursing homes.
For mild illness, antibiotics have not been shown to shorten the duration of symptoms and may make the illness more severe in some people. Severe complications, such as hemolytic uremic syndrome, require hospitalization.
END OF CHAPTER
No comments:
Post a Comment